Technologies for more climate protection
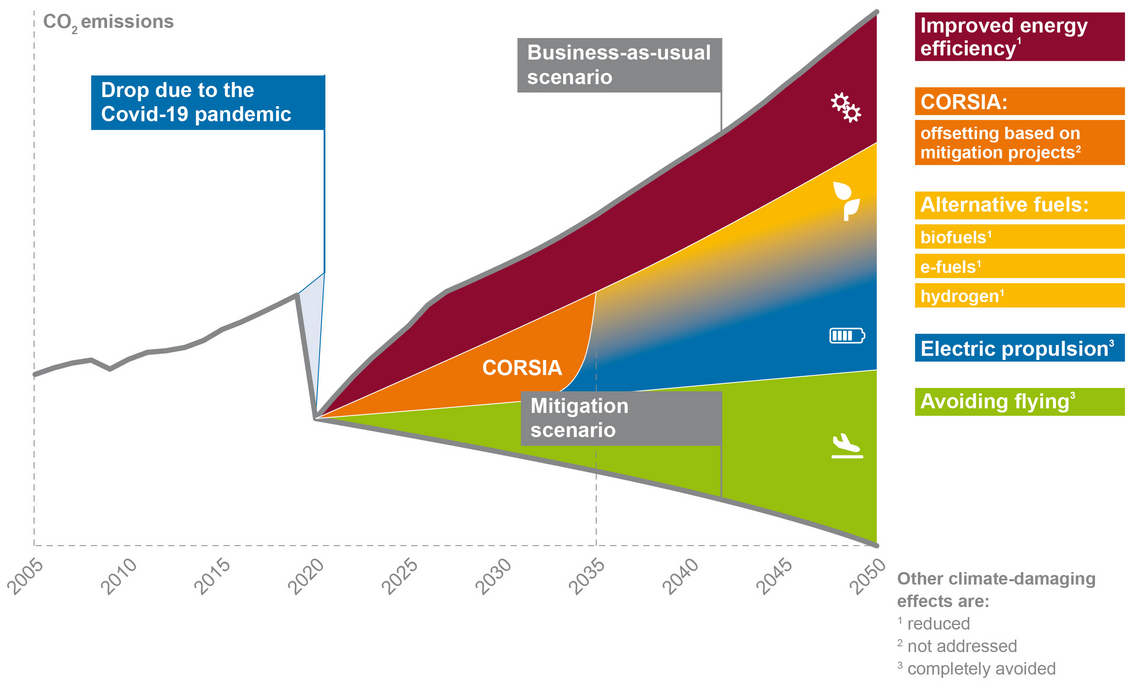
There will continue to be air traffic that cannot be avoided in future. Various measures can be taken to reduce its climate impact and to avoid it as far as possible. In the long term, there are two main ways of doing this: using alternative fuels such as e-fuels (also known as synthetic fuels) or sustainable biofuels; and alternative drive concepts such as hydrogen and electric aircraft.
Regardless of which path is used, increasing energy efficiency also plays a key role. Not only can it decrease specific CO₂ emissions per flight passenger and relieve the high fuel costs of e-fuels, it can also increase the cruising range of aircraft with alternative drive concepts. For all technological options, the decisive factor will be how quickly they become available. The development of new drive systems and aircraft types and of an infrastructure for the supply of hydrogen and for the production of e-fuels require considerable lead times.
The aviation industry does not expect to achieve net zero emissions in their sector before 2060/2065. That’s far too late for the climate. Hence, the avoidance of flights also plays an important role in decarbonizing the sector.
In the medium term – i.e. between 2021 and 2035 – the new instrument of the International Civil Aviation Organization known as CORSIA is also intended to limit some of the emissions from air transport, mainly by buying offset credits. However, the overall contribution of this international instrument to climate protection is far too small. In the long term, offsetting emissions from aviation can no longer play a role. By 2050, we must have transformed our economic systems in such a way that almost no more emissions arise. Natural or technical sinks that absorb CO₂ will be needed to offset the remaining emissions, e.g. from agriculture or industrial processes that cannot be avoided. These sinks, however, are not expected to be available in sufficient quantities to offset emissions from aviation.
Since the total climate impact of aviation is greater than only the effect of CO₂ emissions, the other climate-damaging effects of aviation must also be taken into account in the design of any aviation measures in the future.
Improved energy efficiency
The efficiency can be increased by means of operational and technical measures:
-
Operational measures
Operational measures include, for example, improved airspace management to avoid detours, efficient flight procedures such as a slower descent, climate-oriented optimization of speed and flight profiles, and increased utilization of aircraft capacities.
-
More efficient aircraft engines
Part of the technical measures are, for example, optimized aircraft engines. Today, turbofan engines are the most common type used in commercial aircraft. They produce most of the propulsive thrust not in the main flow, in which air is drawn into the engine and compressed and then used for kerosene combustion, but in the bypass flow which does not pass through the combustion chamber. By increasing the ratio of bypass flow to main flow, fuel consumption can be reduced.
The open rotor concept is seen as a promising option; it has large, externally mounted blade wheels for a bypass flow ratio of 30:1 compared with 11:1 or 12:1 on modern engines. However, the International Energy Agency (IEA) gives it a technology maturity level of 4, which is the designation for an early prototype or a prototype that has proven itself under test conditions. A great deal of development work therefore still needs to be done, e.g. with regard to the higher noise levels. Engines with reduction gears (geared turbofans) are somewhat further along in the development process. They are expected to drive aircraft with a very high bypass ratio of 14:1 to 16:1 and have a lower noise impact than current engines.
-
More efficient aircraft construction
But there are also ways to reduce energy consumption independently of the drive concept. The aerodynamic properties of the aircraft can be improved, for example, by making the surfaces particularly smooth or equipping them with winglets, i.e. curved wingtips, which are already common. Lightweight design can also decrease the weight and thereby fuel consumption. In the future, this can also be achieved by new, aerodynamically optimized aircraft shapes such as the blended wing body (BWB): in this design, the wings and a flattened fuselage, which in turn contributes to lift, merge smoothly.
-
Slow development
At the moment, the efficiency of flying is increasing too slowly. The target set by ICAO to reduce fuel consumption per passenger kilometre by 2% each year is unlikely to be achieved in 2020. Currently, efficiency gains are around 1-1.5%.
Use of alternative fuels
What about the use of already available technologies with “better” fuels? This could become possible with alternative fuels based on biomass and electricity: biofuels from sustainable biogenic sources as well as e-fuels or synthetic fuels produced using renewable electricity.
-
Use of existing infrastructure is possible
Alternative fuels can be blended directly with fossil fuels as drop-in fuels and reduce emissions not only in new aircraft generations, but also in existing aircraft and in conventional aircraft that enter service in the years ahead. This is important because aircraft generally have a long service life of up to 30 years. The use of alternative drop-in fuels is also possible for long-haul flights; the alternative options such as electric and hydrogen propulsion seem unsuitable for the time being.
For the aviation industry, the use of alternative fuels requires only a few changes in infrastructure and operation. Nevertheless, sustainable fuels in aviation account for only 0.05% of all fuels used in the EU. The EU Commission estimates that this share will only increase to a maximum of 2.8% by 2050 if no additional measures are taken to promote these fuels. One important reason for this is that until now, sustainable fuels have been many times more expensive than kerosene.
-
The future of biofuels
It is still uncertain to what extent sustainable biofuels can be used in aviation in the future. Theoretically, it would be possible to cover 100% of the fuel demand with sustainable biofuels produced from energy-rich plant material, agricultural residues such as straw, microalgae or waste such as oils and fats. More than 60% of CO₂ emissions from aviation could be avoided in this way. However, extremely high investments would be needed to establish the infrastructure to produce these fuels. The political framework conditions, such as the design of CO₂ pricing, must also contribute to production of the necessary fuel quantities.
If such fuels were to be used for aviation, this would also exceed the current production of ethanol or biodiesel for road transport. At the same time, huge amounts of water and large areas of land would be needed to grow energy-rich plants. This would conflict with the use of this land for other purposes – additional arable land is essential to grow food and feed a growing world population. Therefore, biofuels will be used only to a limited extent in the near future. They are not a sensible or realistic solution for more sustainable flying
-
What are e-fuels?
E-fuels are similar in chemical composition to fossil fuels such as kerosene. In contrast to those, however, the energy for e-fuels does not come from fossil crude oil, but from electricity. This electricity is used to separate water into its chemical components hydrogen (H2) and oxygen (O2) by means of electrolysis. The hydrogen produced in this way can be used as a basis for further processes.
In the subsequent synthesis process, carbon dioxide and hydrogen are converted into hydrocarbons, which are then processed into fuels, for example. For this, a carbon source is needed in addition to hydrogen. This source, too, must not be fossil in origin so that the fuels have an environmental advantage. CO₂ from biogenic waste gas streams, for example from bioethanol and biogas production, can be used as a sustainable source. These waste gas streams have a very high carbon content, so capture consumes relatively little electricity (0.1 -0.6 megajoules (MJ) per kg CO₂). However, the volume potentials are limited and generation plants are widely distributed across Germany, so this pathway tends to be less suitable for large-scale industrial e-fuel production. Capturing CO₂ from the air is another option in this context, but the energy consumption is significantly higher at 0.7-1.8 MJ (electricity) and 5.4-9.0 MJ (heat) per kg of carbon dioxide due to the low carbon concentration in the atmosphere (0.04%).
-
Advantages and disadvantages of e-fuels
While the volume potential of sustainable biofuels for aviation is considered to be limited, the potential of power-to-liquid is considered to be sufficient in the long term. A draft bill from the German Federal Ministry of the Environment provides for a minimum quota for renewable aviation turbine fuels of non-biogenic origin of 0.5% from 2026. The quota is to rise to 1% by 2028 and 2% by 2030. In addition, measures should be taken to promote the production of these fuels.
It is important to define sustainability criteria at an early stage for fuels produced domestically as well as for those that are imported. These criteria should address:
-
the electricity used in their production and their integration into the power system,
-
the CO₂ source for the production of hydrocarbons, and
-
other social and environmental challenges.
The purchased electricity is the most relevant factor in the environmental impact of electricity-based fuels. This is because if the electricity is generated with fossil fuels, the production of e-fuels can actually bring about more emissions.
A disadvantage of synthetic fuels is the limited greenhouse gas mitigation potential. Although (fossil) CO₂ emissions can be avoided, the non-CO₂ effects of emissions continue to be relevant. Overall, it is estimated that the use of e-fuels reduces the climate impact only by about 30% to 60%.
Furthermore, a huge, additional expansion of renewable energies would be necessary to meet the electricity demand for e-fuels and electric aircraft sustainably. According to calculations made by Oeko-Institut, Germany’s entire renewable electricity production would currently have to be used to replace the amount of kerosene re-fuelled in Germany with sustainably produced e-fuels. However, we still need renewable electricity for many other purposes, e.g. lighting, heat and trains. Globally, the situation is similar.
Hydrogen aircrafts
Aircraft powered by hydrogen could also be a long-term option: Hydrogen can be produced from renewable electricity and could be burned in the aircraft, either in turbines or converted into electricity in a fuel cell that powers the propellers. Hybrid systems that use both turbines and fuel cell systems are also conceivable.
Hydrogen was already being tested in aviation in the 1980s: the Tupolev Tu-155 prototype used liquid hydrogen in a jet engine. Research is focusing again on hydrogen options: since the turn of the millennium, several small aircraft that use fuel cells have been analyzed as prototypes; Airbus has announced that it will launch a hydrogen aircraft capable of covering medium distances of up to 4,000 kilometres by 2035.
-
Technology, temperature and tanks
It makes sense to use hydrogen in liquid form as it has a higher energy density. It is stored at ‑253°C in insulated tanks. Despite insulation, however, boil-off can occur: H2 evaporates, leading to energy losses. Appropriate safety systems are necessary to ensure that boil-off does not pose a danger.
Liquefied hydrogen has a volume-based energy density of about 8.5 megajoules per litre (MJ/l), which is about three quarters less than kerosene (35 MJ/l). Hydrogen storage therefore takes up significantly more space in the aircraft. In addition, the tanks are very heavy; in current prototypes, hydrogen accounts for only 20 % of the total weight of the filled tank. In the future, the tanks would have to be improved so that this ratio can be increased to about 35% to 40%. But even then, hydrogen aircraft are expected to be significantly heavier than aircrafts today. Depending on the aircraft type – e.g. regional or long-haul aircraft – and the drive system, the maximum take-off weight could increase by between 10% and 23%. This in turn has a negative impact on energy consumption, which could increase by over 40% for long-haul aircraft.
-
Potential for reducing emissions
What does the use of hydrogen mean for climate protection? Taking into account the non-CO₂ effects, it is estimated that the use of hydrogen as an aviation fuel can reduce the climate impact by between 50% and 75 %. In the case of use in fuel cells, hydrogen is expected to reduce the climate impact by as much as 75% to 90 %.
-
Technically complex, future unclear
From today’s perspective, the use of hydrogen in aviation is a technically complex solution that is unlikely to be used in aviation before 2035. Exceptions such as the micro airplanes already under development are more likely to be viewed as proof-of-concept. Furthermore, it is unclear from today’s perspective how large the potential for sustainably produced green hydrogen actually is in the medium term. This will make it all the more important in 2050 to avoid a substantial share of flights; and to use this cleaner technology, if it is ready for the market by then, only for flights that cannot be avoided.
Electric aircrafts
Battery electric aircraft are powered by electric engines that draw their energy from rechargeable batteries. Battery electric propulsion could be the most climate-friendly option in aviation: no CO₂ emissions are produced and, unlike alternative fuels and hydrogen-powered aircraft, non-CO₂ effects do not occur. In 2018, Norway – which is the country most advanced in terms of electrification of car transport – announced that all national short-haul flights will be electric by 2040.
A technology in development
The development of battery- powered commercial aircraft and hybrid-electric aircraft is still in its infancy. The International Energy Agency cites a technology maturity level of 4 for battery-electric aircraft and refers to them as an early prototype or a prototype proven under test conditions. Nevertheless, electric propulsion is already being used, e.g. in very small aircraft like the Airbus E-Fan. The first test aircraft with space for up to nine passengers is also in trial operation; commercial use of a converted Cessna is targeted for 2021. However, the possible flight duration and range of 30 minutes and about 160 kilometers are still very low.
The biggest disadvantage of battery electric propulsion compared to kerosene is the low energy density and thus the high weight of the Li-ion batteries. According to the German Aerospace Center, an all-electric A320 would have to be equipped with a 50-ton battery for a 20-minute flight, which is equivalent to one-third of the aircraft’s maximum take-off weight. To power larger aircraft electrically, therefore, a substantially higher energy density of batteries is required.
To tackle these challenges, hybrid concepts are being researched alongside battery-electric aircraft. Airbus, for example, has developed the E-Thrust concept, which, in addition to electric motors, has a conventional jet engine on the rear fuselage to generate additional thrust during take-off. At cruising altitude, the jet engine would be heavily throttled back, generating electricity only to power the aircraft’s electric engines.
From today’s perspective, it seems likely that it will be another 20 to 30 years before electric drives are used on a relevant scale in commercial aircraft. And even then, their use will be limited to smaller aircraft and short distances of at most 500 to 1,000 kilometres – a distance for which rail transport is often well suited. Using battery-electric aircraft for this purpose therefore only makes sense where no rail infrastructure can be built, e.g. for flying on islands
A technology mix for the future
There are therefore several options for making unavoidable aviation more climate-friendly in the future. The options have advantages and disadvantages. However, depending on the option, it will probably take at least 20 to 30 years before they are applied on a relevant scale, either because the technologies are not yet fully developed or the available volume potential of the fuels is not sufficient in the short term.
Bio fuels | E-Fuels | Battery-electric | Hydrogen | |
---|---|---|---|---|
Feeder < 19 seats | no limits on cruising | maximum cruising range of 500-1000 km | no limits on cruising range | |
Regional 20-80 seats | ||||
Short-haul 81-165 seats | ||||
Medium-haul 166-265 seats | n/a | revolutionary aircraft design needed for distances over 10,000 km | ||
Long-haul >250 seats | ||||
Main advantage | drop-in fuel – no or only minor modifications to aircraft and infrastructure necessary | drop-in fuel – no or only minor modifications to aircraft and infrastructure necessary | no climate impact during flight | high GHG mitigation potential (50% to 75% turbine, 75-90% fuel cell) |
Main disadvantage | very limited reduction of non-CO₂ effects | limited reduction of non-CO₂ effects (GHG reduction 30%-60%) | necessary to adapt infrastructure, technology not yet mature | necessary to adapt infrastructure, technology not yet mature |
By 2050, however, the technology mix of electric and hydrogen aircraft for short-haul air transport and alternative fuels for long-haul air transport could help reduce greenhouse gas emissions from unavoidable flights. In addition, to make these flights completely free of emissions, air transport operations could be adapted. For example, lower cruising altitudes could be selected to completely avoid the non-CO₂ effects as well. For non-CO₂ effects that cannot be avoided, the so-called DACCS process (Direct Air Carbon Capture and Storage) could be used to balance emissions. In this process, CO₂ is captured from the air and geologically stored in order to remove it from the atmosphere.
Links
-
Clean Sky 2 JU und FCH 2 JU (2020): Hydrogen-powered aviation - A fact-based study of hydrogen technology, economics, and climate impact by 2050.
-
DLR (2017): Die Zukunft fliegt elektrisch! Vom Lufttaxi bis zum Regionaljet.
-
EASA; EEA; EUROCONTROL (2019): European aviation environmental report 2019.
-
Oeko-Institut (2019): Kein Selbstläufer: Klimaschutz und Nachhaltigkeit durch PtX.
-
Oeko-Institut (2020): E-Fuels im Verkehrssektor.
-
Infras AG; Quantis (2015): Postfossile Energieversorgungsoptionen für einen treibhausgasneutralen Verkehr im Jahr 2050: Eine verkehrsträgerübergreifende Bewertung.
-
UBA (2019): Wege in eine ressourcenschonende Treibhausgasneutralität – RESCUE: Langfassung.
-
UBA (2019): Umweltschonender Luftverkehr lokal – national – international.
-
UBA (2019): Wohin geht die Reise? Luftverkehr der Zukunft: umwelt- und klimaschonend, treibhausgasneutral, lärmarm.